Aero-Structural Optimization of High-Load Carrying Wings for Airborne Wind Energy Application
Airborne Wind Energy (AWE) describes the technology of harvesting wind energy at high altitudes, which cannot be reached by conventional wind turbines. The goal of the AWE project at the CMASLab is to develop high load carrying, light-weight, and shape adaptive wings for AWE applications.
Airborne Wind Energy (AWE) systems are based on an aircraft that reels out a tether connected to a ground-based electric generator. By utilizing a high-lift generating airplane, the wind power is transferred to the generator through the tether, where it is transformed into electrical energy. After the tether is reeled out, the airplane returns towards the ground station, reeling in the tether, and the power-generating phase starts anew.
The airplane can reach higher altitudes than conventional wind turbines, thereby exploiting the stronger and more consistent winds. The control aspects of such systems have been extensively investigated in the past decade. However, the structural and aerodynamic aspects of an AWE aircraft have yet to be optimized, in order to maximize the energy extraction.
This project at CMASLab analyses the structural and aerodynamic behaviour of wings for AWE applications. The aerodynamic forces acting on an AWE aircraft are exceptionally high, resulting in large structural deformations. The project aims to develop wings, which withstand these high aerodynamic loads, while allowing the wings to adapt their shape to control the flight and to maximize the generated power.
You can find more information about the project on the group's webpage.
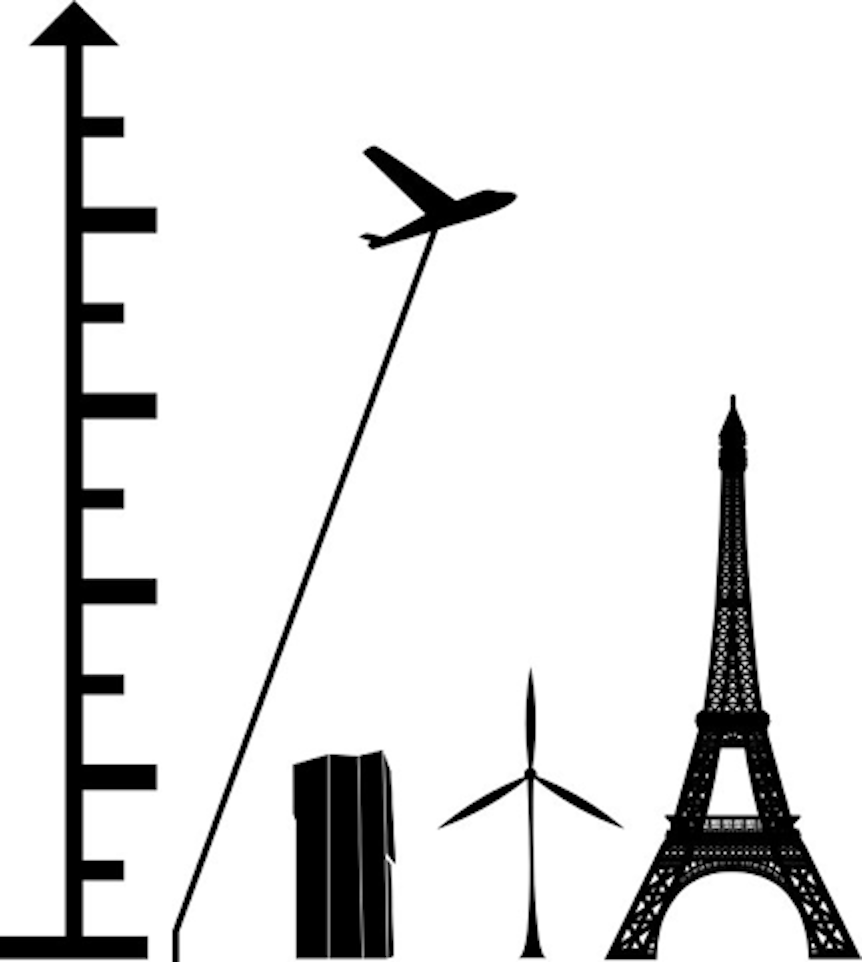
The ESC member involved in this project is Prof. Paolo Ermanni at the Laboratory of Composite Materials and Adaptive Structures (CMASLab) at ETH Zurich.
The group investigates the exploitation of distributed properties of smart materials and structures in the design of novel adaptive systems. Its research is concerned with the exploitation of the distributed properties of structural and smart material components for realising novel behaviours in adaptive structures. They optimise the spatial distribution of structural, actuation and sensing materials for achieving an optimal response across a wide range of operational scenarios and tasks. This is obtained following a highly multi-disciplinary design and optimisation methodology, which allows them to fully exploit the potential of adaptive structures and multi-functionality in applications ranging from: morphing structures, energy harvesting, and highly structurally integrated distributed actuation and sensing systems.